User:Robertinventor/Simple animals could live in Martian brines - Extended Interview with planetary scientist Vlada Stamenković
Now published as Sponges on Mars? We ask Stamenković about their oxygen-rich briny seeps model
Please don't share. For publishing to my own wiki and blog. This article is mid edit. If you spot any errors be sure to say! Robertinventor (talk) 13:24, 15 December 2018 (UTC)
Excluded from search engines
25 October 2019 Vlada Stamenković of the NASA Jet Propulsion Laboratory and colleagues have developed a chemical model of how oxygen dissolves in salty brines in the cold Martian conditions. They found that the trace amounts of oxygen in its thin atmosphere get concentrated into oxygen-rich brines once they reach equilibrium with the atmosphere. Their work suggests that the coldest brines have enough oxygen to support simple animals such as sponges. The model was published in Nature on October 22 2018.
Wikinews caught up with him in an email interview to find out more about his team's research and their plans for the future.
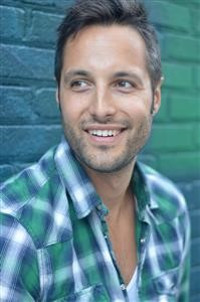
This is an expanded verson of the Wikinews article Simple animals could live in Martian brines: Wikinews interviews Vlada Stamenković which I collaborated on, with more background information, and his answers to some additional questions I asked via email (I'm the volunteer reporter who interviewed him).
Intro[edit | hide | hide all]
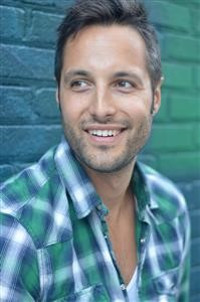
The atmosphere of Mars is far too thin for us to breathe. It would not be possible for lungs like ours to extract oxygen at all. The moisture lining our lungs would boil at well below blood temperature in the thin atmosphere (much like the way that you can't make a good cup of tea at Everest base camp because the water boils at too low a temperature).
The Mars atmosphere has a pressure of only 0.6% of Earth's atmosphere, on average. Also it's mainly carbon dioxide; only 0.146% of that thin atmosphere is oxygen. Yet the result of their modeling was clear. In the cold conditions on Mars these minute amounts of oxygen can get into the salty seeps of water which may be present there. What's more,there's enough oxygen in the brines anywhere on Mars to support microbes that depend on oxygen (aerobes). There's a caveat here; they don't know how long it would take to reach those levels but once it reaches equilibrium with the atmosphere then that's how much oxygen there should be in the brines.
Oxygen permits a more energy intensive metabolism, and many microbes and almost all complex multicellular life on Earth depend on oxygen. They found that cold water would take up much more oxygen than warm water. In the coldest salty water in the polar regions pf Mars, covering perhaps 6.5% of the Martian surface, oxygen levels may be get high enough for simple animals such as sponges. It's a similar picture for the Earth, the tropical oceans, which are warmer, have less oxygen than our polar oceans.
As interviewed by Wikinews:
- VS: Our work really opens up new possibilities for the Martian habitability, and that’s why it’s so exciting!
As previously interviewed by National Geographic (October 22 2018):
- Vlada Stamenković: We were absolutely flabbergasted. I went back to recalculate everything like five different times to make sure it's a real thing.

Some background may help before we get to the main interview with Vlada Stamenković.
If you want to go straight to the interview itself, it's here:
- skip to #Interview
Why salty water?[edit | hide]
(background information): You might wonder why they focus their research on salty solutions. What about fresh water?
Well, fresh water is likely to be rare on present day Mars. Usually the air pressure is so low that it is not stable even at just above freezing, at 0 °C. The Mars atmospheric is thicker at the depths of the huge ancient impact crater of the Hellas basin and other low points on the surface. This raises the boiling point of fresh water to 10 °C, but that means that even there, it is close to boiling point already as soon as it melts at 0 °C. At that temperature, the water would evaporate away rapidly, indeed the pressure there is so low that ice also isn't stable at 0 °C either even in the Hellas basin.
However, salty brines can be liquid at well below 0 °C, for the same reason salt helps keep roads ice free. These salts also counteract the tendency of the water to evaporate at low pressures. Not only that, salts can take in water from the atmosphere too, in the process known as deliquescence, and take up water especially easily at low temperatures.
Curiosity discovered indirect evidence of deliquescence in the equatorial regions (through humidity measurements). These regions are so dry that there is no ice in the surface soil. It is also dry to considerable depth, yet the Curiosity team found that brines form during winter nights in the top 15 cm of the soil when the salts reach temperatures of around -70 °C. The water then evaporates again as the soil warms up through the day, and the process repeats every day - night cycle.
The recurring slope lineae[edit | hide]
There is indirect evidence for other salty brines on Mars, perhaps more habitable than the Curiosity brines. In their paper, Stamenković et al. mention the hydrated magnesium and calcium salts associated with the Recurring Slope Lineae. These seasonal streaks form in spring on sun facing slopes, extend and broaden through the summer and fade away in autumn. The streaks themselves are not damp patches, but they may be associated with thin seeps of brine just below the surface.
Later research suggests that dust flows may also be involved. However the hydrated perchlorate salts observation still has to be explained, as well as the seasonal timing, not correlated with the winds. This is considered to be good evidence that there is at least an element of seasonal hydration associated with the streaks. The literature on this topic has a vigorous dialog between researchers who favour greater or lesser elements of brines in this process.

Significance of oxygen[edit | hide]
Before Vlada Stamenković and his team's new results, scientists assumed any present day Martian life in these and other habitats would have to be able to grow without oxygen. That leaves many possibilities for Martian life include certain blue-green algae such as chroococcidiopsis, some black fungi, and some purple salt loving haloarchaea found in salt ponds and hypersaline lakes on Earth. . Experiments in Mars simulation chambers by DLR in Germany found that lichens such as Pleopsidium chlorophanum may also have some potential to survive in the Mars surface conditions without oxygen. They can do this because the algal component of the lichen is able to make the oxygen needed by its fungal component.
However oxygen rich brines would permit a more energy intensive metabolism and perhaps even true multicellular animal life such as simple sponges. Almost all complex multicellular life uses oxygen.
As previously interviewed by Scientific American (October 22):
- VS: Our work is calling for a complete revision for how we think about the potential for life on Mars, and the work oxygen can do, implying that if life ever existed on Mars it might have been breathing oxygen
Lowest oxygen concentrations[edit | hide]
Note: The paper shows oxygen concentrations in moles per cubic meter. Other sources give the concentrations in miligrams per liter. To convert moles of oxygen per cubic meter to milligrams per litre multiply by 32.
Some aerobic (oxygen using) microbes can survive with as little as a millionth of a mole per cubic meter (0.000032 mg, or 32 nanograms per liter).
Their lowest calculated oxgygen concentrations are for the tropical southern uplands, where temperatures are high and the atmosphere is thin, and for their brine with the lowest oxygen solubilities, sodium perchlorate. They also calculated this figure using their "worst case scenario" - this means, their least optimistic assumptions. However, they give reasons for believing that their more optimistic best case calculations are close to the true situation.
Setting everything to the worst case in this way, they obtained a value of 2.5 millionths of a mole per cubic meter (0.0008 mg per liter).
Levels in the tropical regions would be higher at the lowest points such as the floor of the Hellas basin, south of the equator, where the atmospheric pressure is highest, reaching around 1% of Earth's atmosphere.
Highest oxygen concentrations in their maps[edit | hide]

Since cold brines take up more oxygen, it's no surprise that they found the highest concentrations in polar regions. That's where it reaches the levels high enough for simple sponges.

They base this on another paper from 2014 that showed that some simple sponges can survive with only 0.002 molesper cubic meter (0.064 mg per liter) .
They looked closely at two brines, magnesium and calcium perchlorate, common on Mars. Previous papers show that starting with these brines in a warmer liquid state in Mars simulation experiments, they can be supercooled to temperatures as low as -123 to -133 °C while remaining liquid, before they transform to a glassy state. This supercooling works even when the salty brines are mixed with the martian soil (regolith). It's at these very low temperatures that they will reach the optimal oxygen concentrations.
The oxygen concentrations in calcium perchlorate brines got high enough for simple sponges in regions poleward of about 67.5° to the north and about 72.5° to the south. As you get closer to the poles, oxygen concentrations could go higher, reaching 0.2 moles per cubic meter (6.4 mg per liter). This is not far off levels typical of sea water on Earth.
(background information): on Earth, the most oxygen you can get in warm sea water is about 0.28 moles per cubic meter (9 mg per liter) at 20 °C which increasess to 0.34 moles per cubic meter (11 mg per liter) at 0 °C because cold water takes up the oxygen more readily..
On Earth, worms and clams that live in the muddy sea beds require 1 mg per liter, bottom feeders such as crabs and oysters 3 mg per liter, and spawning migratory fish 6 mg per liter, all within their 0.2 moles (6.4 mg) per liter..
The brine that achieved the highest oxygen solubility is magnesium perchlorates. With this, oxygen concentrations could reach values as high as two moles per cubic meter (64 mg per liter) for the best case with supercooling.
This new research greatly expands the possibilities for complex life on Mars.
Interview[edit | hide]
Could Mars have creatures as active as our worms and fish?[edit | hide]
With this background, Wikinews asked him whether Mars could have life as active as the Earth life that survives at similar oxygen levels

Wikinews
Does your paper's value of up to 0.2 moles of oxygen per cubic meter, the same as Earth's sea water mean that there could potentially be life on Mars as active as our sea worms or even fish?
- VS: Mars is such a different place than the Earth and we still need to do so much more work before we can even start to speculate.
How could life use oxygen at such low temperatures?[edit | hide]
(background information): Earth microbes live in the slow lane at lower temperatures to the point where individual microbes have lifetimes of millennia. Such life is hard to study. It's almost impossible to tell whether it is
- active and able to reproduce at those temperatures
- active and not able to reproduce, or
- intermittently sometimes active and sometimes dormant.
Researchers can't study the reproduction of such slowly growing cells using cell counts. Perhaps it is possible at lower temperatures, but the usual limit cited is -20 °C. That's well above the lowest Martian temperatures studied in the paper of -133 °C.
Is Martian life able to reproduce below these temperatures?
Dirk Schulze-Makuch has proposed that Martian life might evolve an exotic metabolism with the perchlorates of Mars taking the place of the salts inside the cells of Earth life. This would have advantages on Mars, with the brines inside their own cells acting as an anti-freeze to protect them against extreme cold. These salts are also very hygroscopic, which might help life of this sort (if it exists) to scavenge water from the atmosphere and their surroundings.
With this background, Wikinews asked:

WN
The temperatures for the highest levels of oxygen are really low -133 °C, so, is the idea that this oxygen would be retained when the brines warm up to more habitable temperatures during the day or seasonally? Or would the oxygen be lost as it warms up? Or - is the idea that it has to be some exotic biochemistry that works only at ultra low temperatures like Dirk Schulze-Makuch's life based on hydrogen peroxide and perchlorates internal to the cells as antifreeze?
- VS: The options are both: first, cool oxygen-rich environments do not need to be habitats. They could be reservoirs packed with a necessary nutrient that can be accessed from a deeper and warmer region. Second, the major reason for limiting life at low temperature is ice nucleation, which would not occur in the type of brines that we study.
WN
[follow up question] When you talked about warm water encountering the brines from below, in our interview - did you have any thoughts about where the water might come from? Geothermal hot spots?
- VS: That is possible.
(background information): His first suggestion here is that the cool oxygen rich reservoirs could have warmer water come up through them from below. Our orbiting spacecraft have not yet found any subsurface hotspots, but they may be hard to spot from orbit. Mars counts as still geologically active as Olympus Mons has been active as recently as 2.5 million years ago.
So one way to have life there could be that warmer water could rise to the surface from below, heated by the hotspots, and encounter these cold oxygen-rich brines. Then life in the warmer brines could make use of oxygen where the two mix.
The other possibility is that microbes can continue to function at very low temperatures in the Martian conditions. After the interview I discovered that they go into this for their paper in a section "3.2 The lower temperature limit for life and the potential of aerobic habitats" in the supplementary information.
When microbes able to live in extremely cold conditions are cooled down, the interior doesn't freeze but changes to a glassy state (Intracellular vitrification). So the microbes would be able to be cooled down this far without being damaged.
It would then depend on whether the cells can keep their intercellular fluids liquid as they cool down. If they can, this will keep the viscosity low, and permit vigorous metabolic processes to continue. If their interior changes to a glassy state then some metabolic processes do still continue but only very slowly in these vitrified cells.
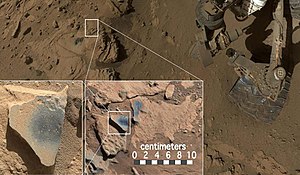
Special minerals on Mars[edit | hide]
(background information): Opportunity discovered manganese oxygens on Mars. These require oxygen to form. Some researchers have suggested they formed in an early Mars atmosphere that was thick and oxygen rich (not necessarily due to life - it could be oxygen rich due to ionizing radiation splitting water).
Their research gives an alternative explanation for these minerals, by concentrating the minute traces of oxygen in the atmosphere into the water.
As previously interviewed by National Geographic (October 22):
- VS: Our explanation doesn't need any special magic — it works on Mars today,
More detailed models[edit | hide]
The paper is theoretical and is based on a simplified general circulation model of the Mars atmosphere - it ignores distinctions of seasons and the day / night cycle. But it takes account of topography (mountains, craters etc) and the axial tilt. They combined it with a chemical model of how oxygen would dissolve in the brines and used this to establish predicted oxygen levels in the brines at the various locations on Mars.
Wikinews asked if they have plans to look into a more detailed model:
WN
and about whether there are any future plans for using a more detailed model with time variation diurnally or seasonally.
- VS: Yes, we are now exploring the kinetics part and want to see what happens on shorter timescales.

(background information): Their model took account of the tilt of the Mars axis, which varies much more than for Earth (our axis is stabilized by the presence of the Moon). They found that for the last five million years conditions were particularly favorable for oxygen rich brines, and that it continues like this for ten million years into the future, as far as they ran the model. For the last twenty million years, as far back as they took their modeling, oases with enough oxygen for sponges are still possible.
WN
What was the resolution for MarsWRF
- VS: Both the horizontal and vertical resolution of the model are variable and selectable at run time; we use a 40-layer vertical grid (0–80 km), following a modified-sigma (terrain-following) coordinate. The lowest model layer with this vertical grid is ~75–100 m above ground level, depending on location and season. We use a horizontal resolution of 5° × 5°, which corresponds to a grid of 72 points in longitude × 36 points in latitude.
Could sponges survive through times when the tilt is higher?[edit | hide]
Remarkably, as they say in the paper, present day Mars would have more oxygen available for life than early Earth had prior to 2.35 billion years ago. On Earth, photosynthesis seems to have come first, before complex multicellular life, generating the oxygen for the first animals. On Mars, with a different source for oxygen, oxygen breathers could arise before photosynthesis. They suggest in their paper that this gives broader opportunities for oxygen-breathing life on other planets.
Wikinews asked Vlada Stamenković if he had any ideas about whether and how sponges could survive through times when the tilt was higher and less oxygen would be available:
WN
I notice from your [4] that there is enough oxygen for sponges only at tilts of about 45 degrees or less. Do you have any thoughts about how sponges could survive periods of time in the distant past when the Mars axial tilt exceeds 45 degrees, for instance, might there be subsurface oxygen rich oases in caves that recolonize the surface? Also what is the exact figure for the tilt at which oxygen levels sufficient for sponges become possible? (It looks like about 45 degrees from the figure but the paper doesn't seem to give a figure for this).
- VS: 45 deg is approx. the correct degree. We were also tempted to speculate about this temporal driver but realized that we still know so little about the potential for life on Mars/principles of life that anything related to this question would be pure speculation, unfortunately.
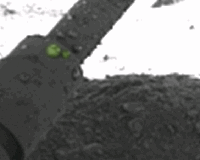
Could oxygen get into rapidly forming droplets where salt forms on ice?[edit | hide]
(background information): When the Phoenix lander landed on Mars in 2008, what appeared to be droplets formed on its legs. They grew, coalesced, and then disappeared, presumably falling off its legs. It was not able to analyze these droplets, but simulations since then by Nilton Renno and his team using the Michigan Mars simulation chamber have shown that such droplets can form within minutes when salt overlays ice on Mars. With this background then Wikinews asked him if he had investigated the timescale, and if so, whether these brines could become oxygenated.
WN
How quickly would the oxygen get into the brines - did you investigate the timescale?
- VS: No, we did not yet study the dynamics. We first needed to show that the potential is there. We are now studying the timescales and processes.
(background information): It is no wonder that this is a challenge. For instance, Curiosity measures temperature changes of around 70 °C between day and night. Also there are large pressure differences between summer and winter. In Gale crater it varied from under 7.5 mbar to nearly 9.5 mbar. There are also large pressure differences between day and night, varying by 10% compared to a tenth of a percent on Earth. On Earth we see such large pressure differences only during a major hurricane.
WN
Could the brines that Nilton Renno and his teams simulated forming on salt / ice interfaces within minutes in Mars simulation conditions get oxygenated in the process of formation? If not, how long would it take for them to get oxygenated to levels sufficient for aerobic microbes? For instance could the Phoenix leg droplets have taken up enough oxygen for aerobic respiration by microbes?
- VS: Just like the answer above. Dynamics is still to be explored. (But this is a really good question 😉).

WN
Any idea of timescale yet for the oxygen to be taken up - is this hours, days, months, years? E.g. could oxygen get into water from melting of morning frosts, and ice melting briefly in the Hellas basin or is this impossible on such a short timescale (if you know)? Similarly for the deliquescing salts that Curiosity found beneath its wheels as it drove over the sand dunes, that form overnight and dry up during the day
- VS: This is still fully under investigation, so there’s unfortunately no info on this yet.
Could oxygen get into the subglacial lakes?[edit | hide]
Wikinews also asked how their research is linked to the recent discovery of possible large subglacial lake 1.5 km below the Martian South Pole found through radar mapping.
WN
Some news stories coupled your research with the subglacial lakes announcement earlier this year. Could the oxygen get through ice into layers of brines such as the possible subglacial lakes at a depth of 1.5 km?
- VS: There are other ways to create oxygen. Radiolysis of water molecules into hydrogen and oxygen can liberate oxygen in the deep and that O2 could be dissolved in deep groundwater. The radiolytic power for this would come from radionuclides naturally contained in rocks, something we observe in diverse regions on Earth.
WN
Could radiolysis take the oxygen concentrations higher than the figures you got?
- VS: This is TBD and would depend on the production rate and the depth where it is produced (~pressure).
(background information): There's research by Möhlmann that suggests that fresh liquid water may form in the Martian polar region a few centimeters below clear ice, a process that happens regularly in Antarctica. If similar clear ice exists on Mars, this process should happen even at very low surface temperatures. Our reporter, referring to this research, asked him:
WN
Could it get into a layer of fresh water just 30 cms below clear ice melted by the solid state greenhouse effect, as in Möhlmann's model (which forms subsurface liquid water at surface temperatures as low as -56 °C).
- VS: See response above.
(background information): So, his answer here is that it could be possible by the same process, radiolysis of the ice through radioactivity in the rocks.
WN
Could undercooled liquid interface water take up oxygen too?
- VS: Yes.
WN
Any idea of timescale yet for the oxygen to be taken up - is this hours, days, months, years? E.g. could oxygen get into water from melting of morning frosts, and ice melting briefly in the Hellas basin or is this impossible on such a short timescale (if you know)? Similarly for the deliquescing salts that Curiosity found beneath its wheels as it drove over the sand dunes, that form overnight and dry up during the day
- VS: This is still fully under investigation, so there’s unfortunately no info on this yet.
Planetary protection issues[edit | hide]
If there are indeed biologically friendly oases dotted throughout the surface of Mars then this could make it harder to sterilize spacecraft sufficiently to explore Mars. They have to be sterilized in order to avoid introducing Earth life to the habitats and so confusing the searches. If the surface of Mars has these oxygen rich habitable brines then it makes the sterilization requirements more stringent. As the Scientific American article suggests, it might be necessary to sterilize robots completely of all micro-organisms, which would drive up the cost of missions to Mars.
Stamenković as interviewed by Scientific American says
- VS: I think there's a sweet spot where we can be curious and we can be explorers and not mess things up, We have to go for that.
(background information): NASA and ESA both have missions that they plan to launch to Mars in 2020 to search for life but both have the search for past life as their main focus. The last and only missions to search for present day "extant" life on Mars were the Viking 1 and 2 missions in the 1970s. Stamenković would like that to change.
As interviewed by Space.com (October 22) he said.
- VS: There is still so much about the Martian habitability that we do not understand, and it's long overdue to send another mission that tackles the question of subsurface water and potential extant life on Mars, and looks for these signals

(background information): There are many such instruments we could send. One example, the "Chemical laptop" or PISCES under development at JPL is shown to the right. A National Academy of Sciences report released 10th October 2018 emphasizes the need to include in situ life detection instruments on future missions:
"The report highlights the need to include in situ detection of energy-starved or otherwise sparsely distributed life such as chemolithotrophic or rock-eating life. In particular, the report found that NASA should focus on research and exploration of possible life below the surface of a planet in light of recent advances that have demonstrated the breadth and diversity of life below Earth’s surface, the nature of fluids beneath the surface of Mars, and the likelihood of life-sustaining geological processes in planets and moons with subsurface oceans."
What would your new instrument TH2OR do?[edit | hide]
Vlada Stamenković is working on a new instrument TH2OR to send to Mars on some potential future mission. It would search for potentially habitable brines deep below its surface using ultra low frequency radio waves. This is a frequency far lower than that of ground penetrating radar, in the range of a fraction of a Hertz up to kilohertz. Wavelengths are measured in kilometers up to tens of thousands of kilometers or more. Wikinews asked him for more details
WN
And I'd also like to know about your experiment you want to send to Mars to help with the search for these oxygenated brines
- VS: We are now developing at “NASA/JPL-California Institute of Technology” a small tool, called TH2OR (Transmissive H2O Reconnaissance) that might one day fly with a yet-to-be-determined mission. It will use low frequency sounding techniques, capable of detecting groundwater at depths down to ideally a few km under the Martian surface, thanks to the high electric conductivity of only slightly salty water and Faraday’s law of induction. Most likely, such a small and affordable instrument could be placed stationary on the planet’s surface or be carried passively or actively on mobile surface assets; TH2OR might be also used in combination with existing orbiting assets to increase its sounding depth. Next to determining the depth of groundwater, we should also be able to estimate its salinity and indirectly its potential chemistry, which is critical information for astrobiology and ISRU (in situ resource utilization).

(background information): Wikinews asked if this device would use natural sources of ultra low frequency radio waves, or if it would use TDEM - a method that involves setting up a current in a loop to generate a sine wave and then suddenly switching it off and observing the radio waves generated by transient eddy currents. The eddy currents have been compared to a smoke ring, they propagate downwards and outwards, a circular current that gets wider as it gets deeper, creating secondary radio waves in a broad band including ultra low frequency waves. The Russian Mars 94 mission, canceled during the break up of USSR, would have flown a TDEM device to Mars.
WN
Does your TH2OR use TDEM like the Mars 94 mission - and will it use natural ULF sources such as solar wind, diurnal variations in ionosphere heating and lightning?
- VS: The physical principle it uses is the same and this has been used for groundwater detection on the Earth for many decades; it’s Faraday’s law of induction in media that are electrically conducting (as slightly saline water is).
However, we will focus on creating our own signal as we do not know whether the EM fields needed for such measurements exist on Mars. However, we will also account for the possibility of already existing fields.
Background information - history of ideas of oxygen breathing life on Mars[edit | hide]
A historical 101 on multicellular life on Mars may be of interest here. First, of course back in the early twentieth century there was much speculation about oxygen breathing multicellular life there, with Lowell even speculating that intelligent Martians built the canals that he thought he could see in his telescope.
By the time of the first orbital robotic space missions to Mars, it was already clear that the atmosphere was far too thin for terrestrial animals, but there was some hope for plant life. However, the early observations from space showed a barren crater covered land and since then the idea of life on Mars has focused mainly on anaerobic microbes and photosynthetic life.
In 2014, however, researchers for DLR, Vera et al surprised many astrobiologists with their experimental result that lichens could potentially grow on present day Mars. This is multicellular life, but only in the form of photosynthetic life able to produce its own oxygen. They took some lichens, such as Pleopsidium chlorophanum that were able to survive in close to Mars-like conditions high up on Antarctic mountain ranges, surviving only on the humidity in the air without any water. These did show promise in Mars simulation chamber experiments. They can do this because the algal component is able to make the oxygen needed by its fungal component. They can also do this without needing any extra water as intermediary, in the experiments they are able to grow in partial shade, using only the night time humidity of the atmosphere itself.
It is also possible to have microscopic multicellular animals that can manage without oxygen at all (sub millimeter sized). There are only three species of these creatures known on Earth, however, and they are not candidates for life on Mars. They are three species of Loricifera, tiny animals about the size of a large amoeba, are able to survive without oxygen in deep extremely salty mud sediments in the Mediterranean.
Background information - why oxygen is so significant for multicellular life[edit | hide]
An oxygen breathing organism can get far more energy from the same amount of food. Without oxygen, the glucose that's the source of energy in Earth organisms can only be broken up into two pyruvate molecules (each of 3 carbon atoms). This process produces two molecules of ATP, the energy molecule that powers our cells. This is a fast reaction, but the amount of energy it produces is limited. This is the energy production method used in sprinter's "fast twitch" white muscle fibers. When Usain Bolt ran his 100 meters sprint he was relying on this chemical process. He wasn't really using much oxygen, mainly it was using up stores of glucose in his fast twitch muscles.
This is not much use if you want to run a marathon however. Luckily for us, if you use oxygen, glucose can also be reduced all the way to carbon dioxide, and in the process 30 or 32 ATP molecules can be produced from a single molecule of glucose. This is the type of energy production used in the "slow twitch" red muscle fibers of a marathon runner, or ultramarathon runner. It lets them run for many miles using little by way of glucose from their food. It amounts to about 100 calories in excess over the resting metabolic calories, depending on body mass, or around 25 grams of glucose per mile, which in turn is derived from glycogen and then fat as the race progresses). In this way a large organism can sustain itself with little by way of food if it relies on oxygen.
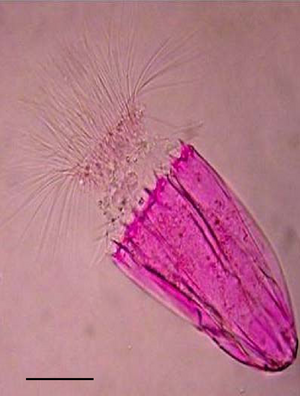
A lifeform that can't use oxygen needs to eat more than ten times as much food to get the same amount of energy as one that can use oxygen. It is hard for it to grow large because so much food is needed just to sustain itself and for reproduction. On Earth we don't know of any anaerobes (organisms that can manage without oxygen) larger than those three species of loricifera, which are less than a millimeter in size.
It is possible for microbes to slowly build large structures without breathing oxygen, as with the stromatolites, which build up layer by layer. However, only the outermost layer, a thin biofilm, is actually alive. Lichens of course do use oxygen, produced by the algal component. Trees and other plants produce oxygen but also use it at night.
What about extraterrestrial life though? Could it use something else in place of oxygen? Well, perhaps, but there is some earlier research that may suggest that it couldn't get very large. In 2005, Catling et al investigated the amount of energy available to carbon based organisms with and without the use of oxygen in their metabolism. Oxygen produces the largest amount of free energy per electron transfer apart from fluorine and bromine, which are too reactive to build up in quantities useful to life. They found on general energetic principles that extraterrestrial life would still needs oxygen to sustain large complex lifeforms, from around 10 cms in size or larger. This is for any extraterrestrial food web for carbon based life.
They worked it out by looking at how many smaller organisms are needed to support larger ones in the food web. For aerobic life the number of organisms in a food web is inversely proportional to the mass, if you are ten times heavier then there are ten times fewer of you in the food web. These numbers are much less for anaerobes.
According to Catling et al's modeling, if an organism is a hundred times larger (and so a million times more massive) in an aerobic food web then it's a million times less numerous (you'd have only one large organism for a million smaller ones).
Meanwhile an organism that's a hundred times larger in an anaerobic food web is a billion times less numerous in an anaerobic food web.
So for instance, if you have a 200 micron organism preying on 20 micron organisms and they in turn on 2 micron organisms then for a billion of the 2 micron organisms there will be a thousand of the 200 micron organisms in an aerobic food chain, but only one in an anaerobic food chain. By the time you get to large organisms of 10 cm scale or larger they should be almost non existent in an anaerobic food web.
If Catling et al are correct in their inference here, then on general energetic principles that an extraterrestrial biosphere with large carbon based animals, at least of 10 cms scale or larger, is going to need oxygen.
Whether or not this applies generally to all extra terrestrial life, it does seem to apply to Earth life. In anoxic environments, Earth animals have found it a challenge to get as large as 1 mm in size without oxygen. It is possible that larger creatures lived on Earth when the seas were all anoxic before the Great Oxygenation Event. There are no surviving multicellular lifeforms from those times but they would be likely to be soft tissued and hard to preserve.
Technical details - guide to paper[edit | hide]
Vlada Stamenković et al's paper explains that their work proceeded by modeling the physics of the solubility of oxygen in brines on Mars. Those were the mixes that permitted the lowest temperatures and so the highest oxygen concentrations. As salts are cooled down, any excess will come out of solution leaving a "eutectic mixture" that has an optimal mixture of water with the salt (e.g. calcium perchlorate) to keep the brines liquid at the lowest possible temperature or eutectic point.
The brines can be cooled down below this theoretical lowest temperature without freezing, in a process known as supercooling. Experiments with Mars soil (regolith) simulants show that even with soil mixed in with the brines, they can still be supercooled to temperatures as low as -123 to -133 °C before transitioning to a glassy state.
They studied oxygen solubility with and without supercooling. They also studied two ways of modeling the physics, a best case, which matches the available data within a few percent, and a worst case simulation which gives a thermodynamic lower limit, however in their Methods section they say that their "best case" is most likely close to the actual situation on Mars.
The main points in their research are summarized in their figure 3 which shows two versions of the map, with and without supercooling. The upper figure is the one with supercooling (note the colour-coding is different for the two maps). The map is for calcium perchlorates and they explain that results are comparable for magnesium perchlorates. The dotted lines in that diagram show the polar limit for sponges. The paper says that 6.5% of the surface area of Mars could have oxygen concentrations suitable for primitive sponges. The white and purple colored regions close to the poles are regions that could have oxygen solubilities similar to Earth's oceans, and the paper says that the polar regions have "the greatest potential to harbor near-surface fluids " at 0.2 moles per cubic meter of dissolved oxygen (6.4 mg / liter). The lowest concentration in their model for their best estimate with supercooling is ~2.5 × 10−5 moles per cubic meter of dissolved oxygen in the tropical southern highlands (0.0008 mg per liter).
Techy aside here, Wikinews asked him about what seems to be a dicrepancy beween Figure 2a and Figure 3.
Wikinews
The paper itself mentions a lower limit of 2.5 * 10-5 moles per cubic meter. But in Figure 2a it looks more like 1.<something> for the lower limit for both the magnesium and calcium suphates. Wondered which is right, or am I misunderstanding something and there is no discrepancy?
- VS: Fig 2 is for an average pressure Pav and Temperature T and Pressure P not being correlated (at a point x on the surface P and T are correlated). Correlated (P,T) are used for Fig 3. Note that the lower limit for perchlorates in Fig 3 is larger than in Fig 2, for correlated (P,T) it is also larger for the other brines, and around lower limit of 2.5 * 10-5 moles per cubic meter.
For the effects of the different types of brine, see their figure 22. This is the one that covers the sodium perchlorate and magnesium perchlorate figures. The brines are colour coded as in figure 1, so sodium perchlorate is black, and Magnesium perchlorate is pink. The lowest number in the abstract of 2.5 millionths of a mole per cubic meter is for the sodium perchlorate black bar in figure 2e. The highest figure of 2 moles per cubic meter is for the magnesium perchnlorates pink bar in figure 2a.
The theory is covered in more detail in the supplementary information. In their figure S1, they start with a theoretical curve for liquid water at these ultra low temperatures - which achieves the maximum solubility if such was possible (of course in reality water would be frozen). The oxygen solubility is reduced by salting out factors which differ for the different salts. These are shown in their figure S2. Magnesium and calcium perchlorate have the two highest salting out factors but to compensate their brines remain liquid at far lower temperatures than potasium or sodium perchlorate. The upshot is that the highest oxygen concentrations can be achieved with magnesium perchlorate, with calcium perchlorate next.
The effects of the variations in the tilt of Mars' axis (obliquity) are summarized in their figure 4. Their figure 4a shows how the oxygen solubility varies with the tilt. The global maximum for supercooling and the best estimate is shown as a red line with purple dots and shows potential for sponges at angles up to around 45 degrees. Then figure 4c shows the effect of the variation in tilt for the last 20 million years and the next ten million years. The supercooling best estimate is shown at the top, the gray shaded boxes are times of atmospheric collapse. They observe in the paper that oxygen solubility levels have been particularly high for the last five million years and will continue in the same way for at least ten million years. They have been high enough for simple sponges for at least twenty million years. You can see how this works from this figure. The paper, discussing oases for simple sponges says that at present they are common poleward of about 67.5° north and about − 72.5° south.
They also make a connection with the recently discovered, and mysterious, seasonal variation in methane. This is about the variation in the background levels of methane, not the methane plumes observed from time to time by Curiosity. The background levels of methane observed by Curiosity over five years vary in a regular seasonal fashion from 0.24 to 0.65 ppbv reaching a maximum towards the end of the Northern hemisphere summer (Curiosity is a few degrees south of the equator at Gale crater). They suggest that at the solubilities they found in the paper, the oxygen could explain some surprising observations such as the highly oxidized phases in Martian rocks and this seasonable variability of methane in Gale crater.
The paper is available to read in its entirety through the link provided on the author's website and the Nature Sharedit sharing initiative.
Sources[edit | hide]
- Robert Perkins. "Model Describing Conditions Under Which Oxygenated Water Could Exist on Mars Challenges Traditional Beliefs About Planet's Habitability (Press release)" — Caltech, October 22, 2018
- Vlada Stamenković, Lewis M. Ward, Michael Mischna & Woodward W. Fischer. "O2 solubility in Martian near-surface environments and implications for aerobic life (Original paper, abstract first page is viewable as a free preview if you click on the purchase link)" — Nature, October 22, 2018
- Vlada Stamenković, Lewis M. Ward, Michael Mischna & Woodward W. Fischer. "Supplementary information provided by authors for: O2 solubility in Martian near-surface environments and implications for aerobic life" — Nature, October 22, 2018
- Robert Perkins. "[ Model Describing Conditions Under Which Oxygenated Water Could Exist on Mars Challenges Traditional Beliefs About Planet's Habitability (Press release)]" — Caltech, October 22, 2018
- Vlada.Stamenkovic, sharing is via Nature Sharedit. "Origins of Life & Habitability - authors website with bibliography - and author shared link to the article" — Habilabs, October 22, 2018
- Maya Wei-Haas. "Salty waters on Mars could host Earth-like life" — National Geographic, October 22, 2018
- Andrew Coates. "Sponges from Mars? Study suggests water on the red planet could support life" — The Conversation, October 22, 2018
- Nola Taylor Redd. "Oxygen-Rich Liquid Water May Exist on Mars" — Scientific American, October 22, 2018
- Mike Wall, Space.com Senior Writer. "Salty Martian Water Could Have Enough Oxygen to Support Life" — Space.com, October 22, 2018
- Andrew Robinson, Media Relations Assistant. "NASA Should Expand the Search for Life in the Universe and Make Astrobiology an Integral Part of its Missions, Says New Report" — National Academy of Science, October 10, 2018
External links[edit | hide]
This is background information for the interview questions and article, not news, but rather the context of pre-existing research, to find out more.
TDEM[edit | hide]
How it works and details of the Mars 94 TDEM, precursor to proposed TH2OR
- Grimm, R.E.. "Low frequency electromagnetic exploration for groundwater on Mars (Abstract gives background on technology used for TH2OR, paper goes into detail and also has some details of the Mars 94 TDEM)" — Journal of Geophysical Research: Planets, 107(E2), 2002
Mars 94 mission
- Andrew LePage. "The Future That Never Came: Mars 94" — Author's website, drewexmachina, March 1, 2015
Proposal to use TDEM for Mars in situ resources same technology as for TH2OR
- Filippini, R., Ottonello, C., Pagnan, S. and Tacconi, G., .. "TDEM for Martian in situ resource prospecting missions (same technology as is used for TH2OR)." — Annals of Geophysics, 46(3)., 2003
Brines and fresh water on Mars[edit | hide]
Phoenix droplets
- "The Salty Tears Of Phoenix Show Liquid Water On Mars (the Phoenix lander observations of what seem to be droplets on its legs, these may be the first spacecraft observations of liquid brines on Mars in 2008-9)" — Mars Daily, Ann Arbor MI (SPX) Mar 19, 2009
Experimental work with the Michigan Mars simulation chamber reproducing the droplets:
- Aaron L. Gronstal. "Liquid Water from Ice and Salt on Mars (Nilton Renno's droplets forming in minutes when salt lies on top of ice in Mars simulation conditions)" — Astrobiology Magazine, Jul 3, 2014
Possiibility for fresh water on Mars beneath ice / snow due to solid state greenhouse effect
- Möhlmann, Diedrich T.F.. "Temporary liquid water in upper snow/ice sub-surfaces on Mars? (Möhlmann solid state greenhouse model)" — Icarus, volume 207, issue 1, pages 140–148, 2010
Big overview review paper on the research into possibilities for brines and fresh water on Mars
- Martínez, G.M. and Renno, N.O.. "Water and brines on Mars: current evidence and implications for MSL (review paper on the possibilities for liquid water on Mars as of 2013)" — Space Science Reviews, 175(1-4), pp.29-51., 2013
Limits for water on Mars, close to boiling point:
- Schulze-Makuch, D. and Houtkooper, J.M., 2010. "Making a Splash on Mars (about how water is unstable over most of Mars and close to boiling point of water in the Hellas basin)" — NASA Science, June 29, 2000
Curiosity's brines discovery - indirect but strong evidence
- Paul Rincon Science editor, BBC News website. "Evidence of liquid water found on Mars (Curiosity's discovery of brines formed by deliquescing salts at around -70 °C in the top 15 cms of the soil below its wheels)" — BBC, April 13, 2015
Probable subglacial lakes near south pole.
- Paul Scott Anderson in Space. "Subsurface lake discovered on Mars?" — Earth & Sky, July 26, 2018
Relevant Lifeforms proposed for Mars[edit | hide]
Possibility of exotic biochemistry using perchlorates and hydrogen peroxide inside cells
- Schulze-Makuch, D. and Houtkooper, J.M., 2010. "A perchlorate strategy for extreme xerophilic life on Mars (This is the research I referred to when asking the question about exotic biochemistry that uses perchlorates inside the cells)" — EPSC Abstracts, 5, pp.EPSC 2010-308., 2010
Lichens as first multicellular lifeforms proposed for Mars in modern times
- "Surviving the conditions on Mars (lichens and blue-green algae tested in Mars simulation chambers)" — DLR (German Aerospace - their equivalent of NASA), April 26, 2012
- de Vera, J.P., Schulze-Makuch, D., Khan, A., Lorek, A., Koncz, A., Möhlmann, D. and Spohn, T.,. "Adaptation of an Antarctic lichen to Martian niche conditions can occur within 34 days. (Mars simulation experiments with the lichen Pleopsidium chlorophanum)" — Planetary and Space Science, 98, pp.182-190., 2014
Black fungi for Mars (not multicellular)
- Kristina Zakharova, Gorji Marzban, Jean-Pierre de Vera, Andreas Lorek & Katja Sterflinger. "Protein patterns of black fungi under simulated Mars-like conditions" — Nature Scientific Reports volume 4, Article number: 5114 (2014), May 29, 2014
Why oxygen is useful for multicellular life[edit | hide]
- "CK-12 Biology Advanced Concepts: 5.18 Aerobic vs. Anaerobic Respiration (good exposition of ATP and slow and fast twitch muscles)" — CK-12 Foundation,
- "How Many Calories Are You Really Burning? (summarizes "Medicine and science in sports and exercise")" — Runner's world,
- "Energy expenditure of walking and running: comparison with prediction equations" — Medicine and science in sports and exercise, 36, pp.2128-2134.,
- Catling, D.C., Glein, C.R., Zahnle, K.J. and McKay, C.P.. "Why O2 Is Required by Complex Life on Habitable Planets and the Concept of Planetary “Oxygenation Time”" — Astrobiology, 5(3), pp.415-438., 2005
Animals that can survive without oxygen[edit | hide]
- Fang, J.,. "Animals thrive without oxygen at sea bottom (about Loricifera the amoeba sized animals which survive without oxygen)" — Nature, 2010
- Mentel, M. and Martin, W.,. "Anaerobic animals from an ancient, anoxic ecological niche" — BMC biology, 8(1), p.32., 2010
Dissolved oxygen requirements for animals[edit | hide]
- Daniel B. Mills, Lewis M. Ward, CarriAyne Jones, Brittany Sweeten, Michael Forth, Alexander H. Treusch, and Donald E. Canfield. "Oxygen requirements of the earliest animals (this is the paper they cite on the low oxygen requirements of some sponges, in this case, the breadcrumb sponge, Halichondria panicea)" — Proceedings of the National Academy of Sciences, 111(11), pp.4168-4172., 2014
- "Dissolved Oxygen (amount of oxygen needed for worms, crabs, fish etc)" — Chesapeake bay program,
- "Dissolved Oxygen (more detailed)" — Fondriest,
Other background information[edit | hide]
Example in situ life experiemnt that could be sent to Mars (one of many):
- "'Chemical Laptop' Could Search for Signs of Life Outside Earth (example of instrument that could be sent to Mars to search for life 'in situ')" — JPL, Nov. 16, 2015
Background to possibilities for life on Mars including discussion of cold limit.
- Rummel, J.D., Beaty, D.W., Jones, M.A., Bakermans, C., Barlow, N.G., Boston, P.J., Chevrier, V.F., Clark, B.C., de Vera, J.P.P., Gough, R.V. and Hallsworth, J.E.. "A new analysis of Mars "Special Regions": findings of the second MEPAG Special Regions Science Analysis Group (Covers limits for Earth life on Mars - and has an overview into research into whether -20 °C is the cold limit of life on Earth. It discusses chaotropic agents, which include perchlorates, which work by disrupting the hydrogen bonding of water molecules and so reduce the minimum temperatures for cell division" — Astrobiology Vol. 14, No. 11, 2014
Methane on Mars:
- Jonathan Amos, BBC Science Correspondent. "Curiosity rover sees seasonal Mars methane swing" — BBC, June 7, 2018
- Webster, C.R., Mahaffy, P.R., Atreya, S.K., Moores, J.E., Flesch, G.J., Malespin, C., McKay, C.P., Martinez, G., Smith, C.L., Martin-Torres, J. and Gomez-Elvira, J.,.. "Background levels of methane in Mars’ atmosphere show strong seasonal variations." — Science, 360(6393), pp.1093-1096., 2018
- Witze, A.. "Mars scientists edge closer to solving methane mystery (an alternative solution)" — Nature, October 25, 2018
Variability of Martian temperature and pressure:
- "Temperature range at Gale crater and Atmospheric pressure at Gale crater (tables)" — Curiosity REMS "weather station on Mars", sol 0-179
- Gisela Speidel. "Professor says NASA's Martian weather reports show extreme pressure swings" — University of Hawaiʻi at Mānoa, Sep 24, 2012
Manganese dioxides, evidence of oxygen rich water on Mars in the past
- "NASA Rover Findings Point to a More Earth-like Martian Past (about the manganese oxides)" — JPL, June 27, 2016
Evidence that it is still geologically active
- G. Neukum, R. Jaumann, H. Hoffmann, E. Hauber, J. W. Head, A. T. Basilevsky, B. A. Ivanov, S. C. Werner, S. van Gasselt, J. B. Murray, T. McCord & The HRSC Co-Investigator Team. "Recent and episodic volcanic and glacial activity on Mars revealed by the High Resolution Stereo Camera (evidence that Olympus Mons had volcanic activity as recent as 2 million years ago showing that Mars is still geologically active)" — Nature, December 23, 2004
CAT:SpaceCAT:MarsCAT:NASA CAT:BiologyCAT:Science and technology